

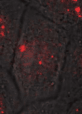
The goal of the Reyes lab is to capitalize on recent developments in fluorescence microscopy to understand DNA replication and genome integrity. Our group pioneers the development of new microscopy, molecular biology and genetic approaches to understand how the sequence in our genome is inherited and preserved by studying live cells. We obtain a broad perspective of the biological problems studied by exploring the diversity of mechanisms developed by life using as model systems the bacterium Escherichia coli, budding yeast and human cells.
Critical to our work is the development and use of live cell single-molecule fluorescence microscopy to understand protein function. Using single-molecule microscopy we study the interaction of the protein of interest with other proteins or with DNA, measure binding kinetics, determine stoichiometries of complexes, and obtain estimates of protein concentrations in cells. Microscopy approaches are complemented in our group by the development of computer scripts for image analysis.
Preserving the genome is a fundamental task in life. Through our work, we aim to contribute in the search of new antibiotic targets, the understanding and treatment of cancer and other genetic diseases, the development of biotechnological applications, and the search of life in the universe.